|
Abbe
number |
 |
A numerical value indicating the
dispersion of optical glass, using the Greek symbol v.
Also called the optical constant. The Abbe number is
determined by the following formula using the index of
refraction for three Fraunhofer's lines: F
(blue), d (yellow) and c (red).
An optical glass characteristic
distribution chart is a graph using the Abbe number as
the horizontal axis and the d line index of
refraction as the vertical
axis.
| |
|
|
Aberration |
 |
The image formed by an ideal
photographic lens would have the following
characteristics:
1. A point would be formed
as a point. 2. A plane (such as a wall)
perpendicular to the optical axis would be formed as a
plane. 3. The image formed by the lens
would have the same shape as the subject.
Also, from the standpoint of image
expression, a lens should exhibit true color
reproduction. If only light rays entering the lens close
to the optical axis are used and the light is
monochromatic (one specific wavelength), it is possible
to realize virtually ideal lens performance. With real
photographic lenses, however, where a large aperture is
used to obtain sufficient brightness and the lens must
converge light not only from near the optical axis but
from all areas of the image, it is extremely difficult
to satisfy the above-mentioned ideal conditions due to
the existence of the following obstructive factors:
• Since most lenses are
constructed solely of lens elements with spherical
surfaces, light rays from a single subject point are not
formed in the image as a perfect point. (A problem
unavoidable with spherical
surfaces.) • The focal point position
differs for different types (i.e., different
wavelengths) of light. • There are many
requirements related to changes in angle of view
(especially with wide-angle, zoom and telephoto
lenses).
The general term used to describe the
difference between an ideal image and the actual image
affected by the above factors is "aberration." Thus, to
design a high-performance lens, aberration must be
extremely small, with the ultimate objective being to
obtain an image as close as possible to the ideal image.
Aberration can be broadly divided into two
classifications: chromatic aberrations, which occur due
to differences in wavelength, and monochromatic
aberrations, which occur even for a single wavelength.
|
Achromat, achromatic
lens |
 |
A lens which corrects chromatic
aberration for two wavelengths of light. When referring
to a photographic lens, the two corrected wavelengths
are in the blue-violet range and yellow range.
|
AF Stop
feature |
 |
Another feature unique to Canon's
four Image Stabilized super-telephoto lenses. Four
buttons appear on the outer barrel near the front of
these lenses; pushing any one will temporarily lock AF
if the camera is in the AI Servo AF mode. Custom
Functions on many newer EOS bodies allow these buttons
to assume a variety of additional functions. |
Air lens |
 |
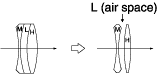 |
Air Lens Concept
Diagram |
The air spaces between the glass lens
elements making up a photographic lens can be thought of
as lenses made of glass having the same index of
refraction as air (1.0). An air space designed from the
beginning with this concept in mind is called an air
lens. Since the refraction of an air lens is opposite
that of a glass lens, a convex shape acts as a concave
lens and a concave shape acts as a convex lens. This
principle was first propounded in 1898 by a man named
Emil von Hoegh working for the German company Goerz.
|
Angle of
view |
 |
The area of a scene, expressed as an
angle, which can be reproduced by the lens as a sharp
image. The nominal diagonal angle of view is defined as
the angle formed by imaginary lines connecting the lens’
second principal point with both ends of the image
diagonal (43.2mm). Lens data for EF lenses generally
includes the horizontal (36mm) angle of view and
vertical (24mm) angle of view in addition to the
diagonal angle of view |
Angular
aperture |
 |
The angle between the subject point
on the optical axis and the diameter of the entrance
pupil, or the angle between the image point on the
optical axis and the diameter of the exit pupil.
|
Aperture / effective
aperture |
 |
The aperture of a lens is related to
the diameter of the group of light rays passing through
lens and determines the brightness of the subject image
formed on the focal plane. The optical aperture (also
called the effective aperture) differs from the real
aperture of the lens in that it depends on the diameter
of the group of light rays passing through the lens
rather than the actual lens diameter. |
Aperture
ratio |
 |
A value used to express image
brightness, calculated by dividing the lens’ effective
aperture (D) by its focal length (f). Since the value
calculated from D/f is almost always a small decimal
value less than 1 and therefore difficult to use
practically, it is common to express the aperture ratio
on the lens barrel as the ratio of the effective
aperture to the focal length, with the effective
aperture set equal to 1. (For example, the EF 85mm
f/1.2L lens barrel is imprinted with 1:1.2, indicating
that the focal length is 1.2 times the effective
aperture when the effective aperture is equal to 1.) The
brightness of an image produced by a lens is
proportional to the square of the aperture ratio. In
general, lens brightness is expressed as an F number,
which is the inverse of the aperture ratio (f/D).
|
Apochromat, apochromatic
lens |
 |
A lens which corrects chromatic
aberration for three wavelengths of light, with
aberration reduced to a large degree particularly in the
secondary spectrum. EF super-telephoto lenses are
examples of apochromatic lenses. |
Aspherical
lens |
 |
Photographic lenses are generally
constructed of several single lens elements, all of
which, unless otherwise specified, have spherical
surfaces. Because all surfaces are spherical, it becomes
especially difficult to correct spherical aberration in
large-aperture lenses and distortion in super-wide-angle
lenses. A special lens element with a surface curved
with the ideal shape to correct these aberrations, i.e.,
a lens having a free-curved surface which is not
spherical, is called an aspherical lens. The theory and
usefulness of aspherical lenses have been known since
the early days of lens making, but due to the extreme
difficulty of actually processing and accurately
measuring aspherical surfaces, practical aspherical lens
manufacturing methods were not realized until fairly
recently. The first SLR photographic lens to incorporate
an aspherical lens was Canon's FD 55mm f/1.2AL released
in March 1971. (Leica offered the 50mm f/1.2 Noctilux
lens with aspherical surfaces for its rangefinder
cameras many years before 1971.)
Due to revolutionary advances in
production technology since that time, Canon's current
EF lens group makes abundant use of various aspherical
lens types such as ground and polished glass aspherical
lens elements, ultra-precision glass molded (GMo)
aspherical lens elements, composite aspherical lens
elements and replica aspherical lens elements.
|
Chromatic aberration
|
 |
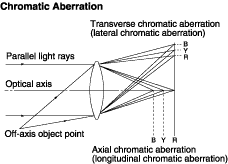 |
When white light (light containing
many colors uniformly mixed so that the eye does not
sense any particular color and thus perceives the light
as white) such as sunlight is passed through a prism, a
rainbow spectrum can be observed. This phenomenon occurs
because the prism's index of refraction (and rate of
dispersion) varies depending on the wavelength (short
wavelengths are more strongly refracted than long
wavelengths). While most visible in a prism, this
phenomenon also occurs in photographic lenses, and since
it occurs at different wavelengths is called chromatic
aberration. There are two types of chromatic aberration:
"axial chromatic aberration," where the focal point
position on the optical axis varies according to the
wavelength, and "chromatic difference of magnification,"
where the image magnification in peripheral areas varies
according to the wavelength. In actual photographs,
axial chromatic aberration appears as color blur or
flare, and chromatic difference of magnification appears
as color fringing (where edges show color along their
borders). Chromatic aberration in a photographic lens is
corrected by combining different types of optical glass
having different refraction and dispersion
characteristics. Since the effect of chromatic
aberration increases at longer focal lengths, precise
chromatic aberration correction is particularly
important in super-telephoto lenses for good image
sharpness. Although there is a limit to the degree of
correction possible with optical glass, significant
performance improvements can be achieved using man-made
crystal such as fluorite or UD glass. Axial chromatic
aberration is also sometimes referred to as
"longitudinal chromatic aberration" (since it occurs
longitudinally with respect to the optical axis), and
chromatic difference of magnification can be referred to
as "lateral chromatic aberration" (since it occurs
laterally with respect to the optical axis). Note:
While chromatic aberration is most noticeable when using
color film, it affects black-and-white images as well,
appearing as a reduction in sharpness. |
Circle of
confusion |
 |
Since all lenses contain a certain
amount of spherical aberration and astigmatism, they
cannot perfectly converge rays from a subject point to
form a true image point (i.e., an infinitely small dot
with zero area). In other words, images are formed from
a composite of dots (not points) having a certain area,
or size. Since the image becomes less sharp as the size
of these dots increases, the dots are called “circles of
confusion.” Thus, one way of indicating the quality of a
lens is by the smallest dot it can form, or its “minimum
circle of confusion.” The maximum allowable dot size in
an image is called the “permissible circle of
confusion.” |
Circular
aperture |
 |
Certain Canon lenses feature a new
Circular Aperture diaphragm unit, which uses curved
aperture blades to provide for a more rounded opening as
the lens is stopped down. It's especially effective at
rendering out of focus background highlights as natural
rounded shapes. In lenses such as the EF 70-200mm f/2.8L
IS lens, the lens opening is virtually circular from
f/2.8 to f/5.6. These lenses retain all the benefits
previously available with Canon's Electromagnetic
Diaphragm – smooth and consistent stop-down operation
(even at up to 10fps with the EOS-1v), near-silent
aperture control, and total absence of mechanical levers
or switches in the lens mount. |
Circular polarizing
filter |
 |
A circular polarizing filter is
functionally the same as a linear polarizing filter as
it only passes light vibrating in a certain direction.
However, the light passing through a circular polarizing
filter differs from light passing through a linear
polarizing filter in that the vibrational locus rotates
in a spiral pattern as it propagates. Thus, the effect
of the filter does not interfere with the effect of
half-mirrors: allowing normal operation of TTL-AE and AF
functions. When using a polarizing filter with an EOS
camera, be sure to always use a circular polarizing
filter. The effectiveness of a circular polarizing
filter in eliminating reflected light is the same as
that of a linear polarizing filter. |
Coating |
 |
When light enters and exits a lens,
approximately 5% of the light is reflected back at each
lens-air boundary due to the difference in index of
refraction. This not only reduces the amount of light
passing through the lens but can also lead to repeating
reflections which can cause unwanted flare or ghost
images. To prevent this reflection, lenses are processed
with a special coating. Basically this is carried out
using vacuum vapor deposition to coat the lens with a
thin film having a thickness l/4 the wavelength of the
light to be affected, with the film made of a substance
(such as magnesium fluoride) which has an index of
refraction of n, where n is
the index of refraction of the lens glass. Instead of a
single coating affecting only a single wavelength,
however, EF lenses feature a superior multi layer
coating (multiple layers of vapor deposited film
reducing the reflection rate to 0.2-0.3%) which
effectively prevents reflections of all wavelengths in
the visible light range. Lens coating is carried out not
only to prevent reflections, however. By coating the
various lens elements with appropriate substances having
different properties, coating plays an important role in
providing the overall lens system with optimum color
balance characteristics. |
Color balance
|
 |
The color reproduction fidelity of a
photo taken through a lens compared to the original
subject. Color balance in all EF lenses is based on ISO
recommended reference values and maintained within a
strict tolerance range that is tighter than ISO's CCI
allowable value range. |
Coma, comatic
aberration |
 |
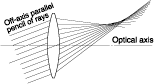 |
Coma, or comatic aberration, is a
phenomenon visible in the periphery of an image produced
by a lens which has been corrected for spherical
aberration, and causes light rays entering the edge of
the lens at an angle to converge in the form of a comet
instead of the desired point, hence the name. The comet
shape is oriented radially with the tail pointing either
toward or away from the center of the image. The
resulting blur near the edges of the image is called
comatic flare. Coma, which can occur even in lenses
which correctly reproduce a point as a point on the
optical axis, is caused by a difference in refraction
between light rays from an off-axis point passing
through the edge of the lens and the principal light ray
from the same point passing through the lens center.
Coma increases as the angle of the principal ray
increases, and causes a decrease in contrast near the
edges of the image. A certain degree of improvement is
possible by stopping down the lens. Coma can also cause
blurred areas of an image to flare, resulting in an
unpleasing effect. The elimination of both spherical
aberration and coma for a subject at a certain shooting
distance is called aplanatism, and a lens corrected as
such is called an aplanat. |
Contrast |
 |
The degree of distinction between
areas of different brightness levels in a photograph,
i.e., the difference in brightness between light and
dark areas. For example, when the reproduction ratio
between white and black is clear, contrast is said to be
high, and when unclear, contrast is said to be low. In
general, quality lenses producing high quality images
have both high resolution and high contrast. |
Cos4 law |
 |
States that light fall-off in
peripheral areas of the image increases as the angle of
view increases, even if the lens is completely free of
vignetting. The peripheral image is formed by groups of
light rays entering the lens at a certain angle with
respect to the optical axis, and the amount of light
fall-off is proportional to the cosine of that angle
raised to the fourth power. As this is a law of physics,
it cannot be avoided. However, with wide-angle lenses
having a large angle of view, decreases in peripheral
illumination can be prevented by increasing the lens’
aperture efficiency (ratio of the area of the on-axis
entrance pupil to the area of the off-axis entrance
pupil). |
Curvature of
field |
 |
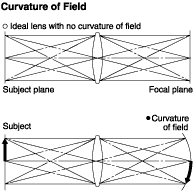 |
Curvature of field is a phenomenon
which causes the image formation plane to become curved
like the inside of a shallow bowl, preventing the lens
from producing a flat image of a flat subject. When the
center of the image is in focus, the periphery is out of
focus, and when the periphery is in focus, the center is
out of focus. The degree of curvature of field is
largely affected by the method used for correcting
astigmatism. Since the image plane falls between the
sagittal and meridional image surfaces, good correction
of astigmatism results in small curvature of field.
Since curvature of field cannot be improved very much by
stopping down the lens, lens designers reduce it as much
as possible using various methods such as changing the
shapes of the various single lens elements making up the
lens and changing the position of the aperture. In doing
this, one necessary condition that must be satisfied to
simultaneously correct astigmatism and curvature of
field is Petzval’s Condition (1843). Petzval’s Condition
states that a lens element is good if a result of zero
is obtained when the inverse of the product of the index
of refraction and focal length of that lens element is
added to the total number of lens elements making up the
lens. This sum is called Petzval’s Sum. |
 |
 |
 | |
| |